What is biological treatment of wastewater & how it works?
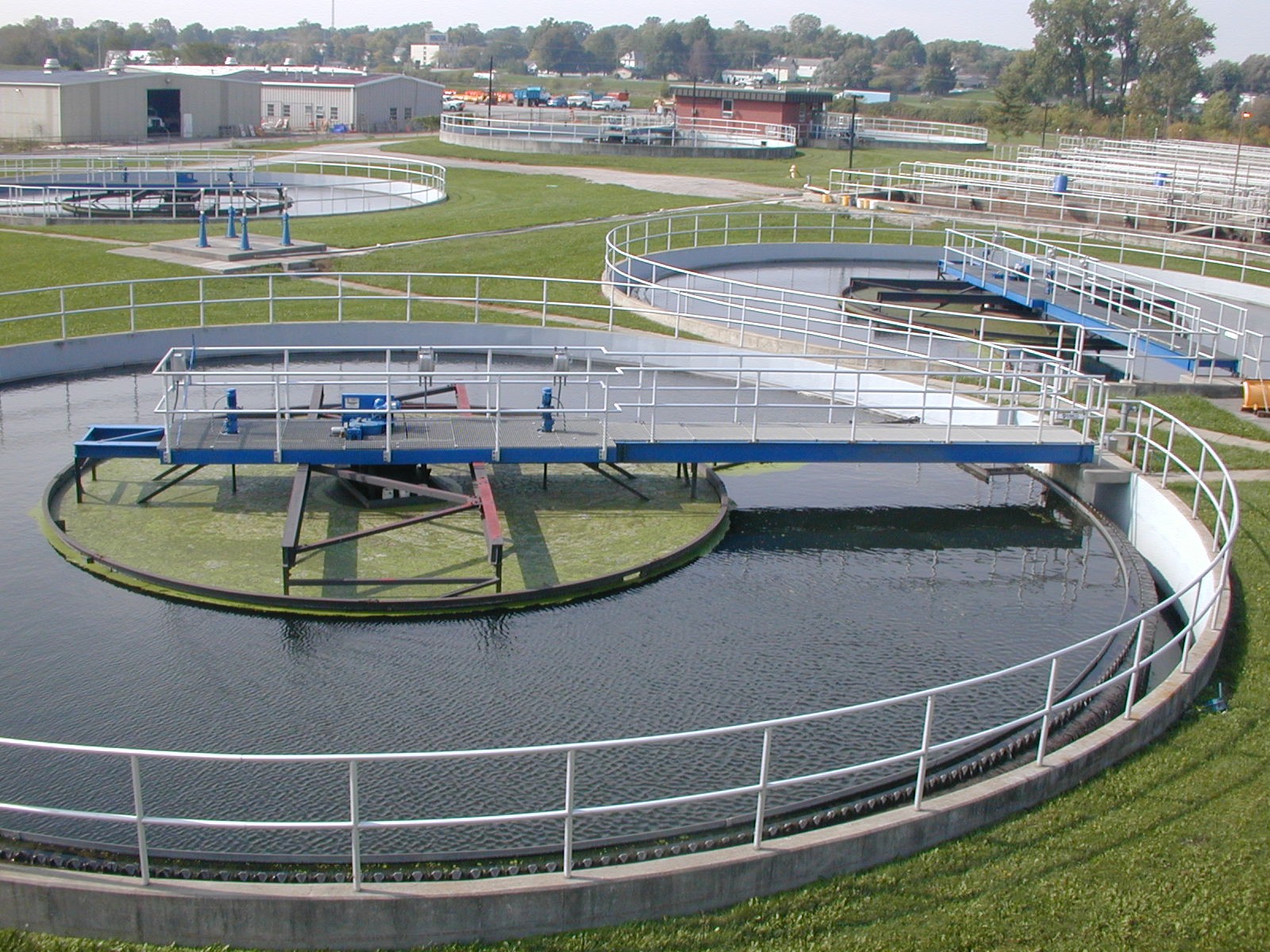
Role of Fermenting Bacteria in Waste Water Treatment – The mixture of domestic sewage, industrial effluent and ground water which has infiltrated into the sewers is present in Wastewater which is 99.99% water, with a small amount of dissolved or suspended solid matter.
One of the applications of fermentation process is in the treatment of wastewater. In the process of sewage disposal, sewage is digested by enzymes secreted by bacteria. Solid organic matters are broken down into harmless, soluble substances and carbon dioxide. The digested solids, also known as sludge, accumulated in a wastewater treatment process must be treated and disposed of in a safe and effective manner. The purpose of digestion is to reduce the amount of organic matter and the number of disease-causing microorganisms present in the solids.
Biological treatment of waste can be carried out aerobically, process known as composting or anaerobically through anaerobic digestion. The degradable organic compounds e.g. carbohydrates, proteins, fats, etc. in the waste water are broken down by aerobic micro-organisms mainly bacteria and rarely fungi. The result is an effluent with drastically reduced organic matter content. The materials difficult to digest form a sludge is treated separately either by aerobic breakdown of raw waste-water or anaerobic breakdown of sludge.
Aerobic Digestion of Raw Waste Water – The most common method involve in aerobic breakdown of raw waste water is the activated sludge system. Activated sludge system involves the production of an activated mass of micro-organisms capable of stabilizing the organic content of a waste aerobically.
Waste water is introduced into an aerated tank of micro-organisms which are collectively referred to as activated sludge or mixed liquor. Aeration is achieved by the use of submerged diffused or surface mechanical aeration which maintain the activated sludge in suspension.
A wide range of bacteria are involved, including Pseudomonas, Lactobacillus and Yeast.
Microorganisms have to cope with an uncontrollably diverse range of organic and inorganic compounds some of which may be toxic to the organisms. The microorganisms occur in discreet aggregates known as flocs. Some of the settled biomass is recycled as ‘returned activated sludge’ inoculate the incoming raw sewage because it contains a community of organisms adapted to the incoming sewage. The solid undigested sludge may be further treated into economically valuable products.
Mode of Action – Nitrification & Denitrification Bacteria remove nitrogen from wastewater by a two step biological processes: nitrification followed by denitrification. Technically, it is a three step process: Ammonification precedes nitrification and denitrification.
Ammonification – While traveling through sewer pipes, the majority of the nitrogen contained in raw sewage (urea and fecal material) is converted from organic-nitrogen to ammonia through a process called hydrolysis. Technically, in the majority of situations, more ammonium than ammonia is created during ammonification. The actual ratio is influenced by pH and temperature.
Nitrification by Nitrifying Bacteria – Nitrification process performed by nitrifying bacteria such as Nitromonas in aerobic treatment
The biological conversion of ammonia to nitrate nitrogen is called Nitrification. Nitrification is a two-step process. Bacteria known as Nitrosomonas convert ammonia and ammonium to nitrite. Next, bacteria called Nitrobacter finish the conversion of nitrite to nitrate. The reactions are generally coupled and proceed rapidly to the nitrate form; therefore, nitrite levels at any given time are usually low. These bacteria known as “nitrifiers” are strict “aerobes,” meaning they must have free dissolved oxygen to perform their work. Nitrification occurs only under aerobic conditions at dissolved oxygen levels of 1.0 mg/L or more. At dissolved oxygen (DO) concentrations less than 0.5 mg/L, the growth rate is minimal. Nitrification requires a long retention time, a low food to microorganism ratio (F:M), a high mean cell residence time (measured as MCRT or Sludge Age), and adequate buffering (alkalinity). The nitrification process produces acid. The optimum pH for Nitrosomonas and Nitrobacter is between 7.5 and 8.5; most treatment plants are able to effectively nitrify with a pH of 6.5 to 7.0. Nitrification stops at a pH below 6.0. The nitrification reaction consumes 7.1 mg/L of alkalinity as CaCO3 for each mg/L of ammonia nitrogen oxidized. An alkalinity of no less than 50-100 mg/L is required to insure adequate buffering. Water temperature also affects the rate of nitrification. Nitrification reaches a maximum rate at temperatures between 30 and 35 degrees C. At temperatures of 40o C and higher, nitrification rates fall to near zero.
At temperatures below 20 degrees C, nitrification proceeds at a slower rate, but will continue at temperatures of 10 degrees C and less. However, if nitrification is lost, it will not resume until the temperature increases to well over 10 o C. Some of the most toxic compounds to nitrifiers include cyanide, thiourea, phenol and heavy metals such as silver, mercury, nickel, chromium, copper and zinc. Nitrifying bacteria can also be inhibited by nitrous acid and free ammonia.
NH3 + O2 → NO2 + 3H+ + 2e−
NH3 + O2 + 2H+ + 2e− → NH2OH + H2O
Denitrification by Denitrifying Bacteria – The biological reduction of nitrate (NO3) to nitrogen gas (N2) by facultative heterotrophic bacteria is called Denitrification. “Heterotrophic” bacteria need a carbon source as food to live. “Facultative” bacteria can get their oxygen by taking dissolved oxygen out of the water or by taking it off of nitrate molecules.
Denitrification occurs when oxygen levels are depleted and nitrate becomes the primary oxygen source for microorganisms. The process is performed under anoxic conditions, when the dissolved oxygen concentration is less than 0.5 mg/L, ideally less than 0.2. When bacteria break apart nitrate (NO3 – ) to gain the oxygen (O2 ), the nitrate is reduced to nitrous oxide (N2 O), and, in turn, nitrogen gas (N2 ). Since nitrogen gas has low water solubility, it escapes into the atmosphere as gas bubbles. Free nitrogen is the major component of air, thus its release does not cause any environmental concern.
The formula describing the nitrification reaction follows:
Optimum pH values for denitrification are between 7.0 and 8.5.
Since denitrifying bacteria are facultative organisms, they can use either dissolved oxygen or nitrate as an oxygen source for metabolism and oxidation of organic matter. If dissolved oxygen and nitrate are present, bacteria will use the dissolved oxygen first. That is, the bacteria will not lower the nitrate concentration. Denitrification occurs only under anaerobic or anoxic conditions.
Another important aspect of denitrification is the requirement for carbon; that is, the presence of sufficient organic matter to drive the denitrification reaction. Organic matter may be in the form of raw wastewater, or supplemental carbon. Conditions that affect the efficiency of denitrification include nitrate concentration, anoxic conditions, presence of organic matter, pH, temperature, alkalinity and the effects of trace metals. Denitrifying organisms are generally less sensitive to toxic chemicals than nitrifiers, and recover from toxic shock loads quicker than nitrifiers. Temperature affects the growth rate of denitrifying organisms, with greater growth rate at higher temperatures. Denitrification can occur between 5 and 30o C, and these rates increase with temperature and type of organic source present. The highest growth rate can be found when using methanol or acetic acid. A slightly lower rate using raw wastewater will occur, and the lowest growth rates are found when relying on endogenous carbon sources at low water temperatures. Wastewater cannot be denitrified unless it is first nitrified.
Use of Fermented Sludge in Nutrient Removal – Fermentation can be used to produce carbon source for both nitrogen and phosphorus removal. It is a tool to reduce operational costs associated with biological nutrient removal. Removal of nitrogen and phosphorus from wastewater effluents is important to ensure environmental protection of surface waters. High concentrations of nutrients in rivers can cause eutrophication, oxygen depletion in the river waters and can stimulate algae growth. Thus it has been proposed as an efficient process to remove nutrients from wastewater.
Biological Nitrogen Removal – The processes for biological nitrogen removal can be incorporated into both activated sludge and percolating filter plants. The overall mechanism follows the route of nitrification (oxidation of ammonia to nitrite and nitrate) and denitrification (reduction of nitrate sequentially to nitrite, nitric oxide, nitrous oxide and nitrogen)]
Biological denitrification processes generally recirculate nitrified effluent as a source of nitrate. This means that 100% removal can never be achieved. Only the nitrate contained in the recirculated stream will be removed.
Biological Phosphorus Removal – Biological phosphorus removal is dependent mainly on the ability of the bacterium Acinerobacter spp. to release phosphate under anaerobic conditions and to absorb it under aerobic conditions.
The mechanism of Phosphorus removal by Acinetobacter spp. includes the following steps:
Under anaerobic conditions, readily biodegradable organic matter becomes fermented to short chain fatty acids. These are stored in the cell as poly- hydroxyl butyrates (PHB).
Under aerobic conditions the stored PHB is oxidized and energy is released allowing the assimilation of soluble ortho-phosphate. The ortho-phosphate is metabolized by the cell and excess quantities are stored in the cell as poly-phosphate. The storage of excess phosphate is known as ‘luxury uptake’ of Phosphorus and it is this particular ability of the cell that is exploited in the nutrient removal process.
Anaerobic Digestion of Sludge – The commonest method of treating sludge however is by anaerobic digestion. This can be done by allowing the sludge to decompose in digesters under controlled conditions for several weeks. Digesters themselves are closed tanks with provision for mild agitation, and the introduction of sludge and release of gases. About 50% of the organic matter is broken down to gas, mostly methane.
Anaerobic Microbial Digestion usually involves four steps:
- Extracellular Hydrolysis (e.g., Cellulose)
- Fermentation leading to organic acids (VFAs), acetate, CO2 and H2
- Fermentation leading to acetic acid (CH3COOH) , H2 and O
- Methanogenesis leading to CH4, CO2 and H2
The fermentation process occurs in two main steps: hydrolysis and acidogenesis. During hydrolysis the long chain molecules are broken down into smaller dissolved molecules by extracellular enzymes that are then converted to VFAs during the acidogenesis step.
First, during hydrolysis, water-insoluble biopolymers such as carbohydrates, proteins, and fats are decomposed by extracellular enzymes to water-soluble monomers (e.g., monosaccharide’s, amino acids, glycerin, fatty acids) and thus made accessible to further degradation.
In the second step (acidification) the intermediates of hydrolysis are converted into acetic acid (CH3COOH), hydrogen (H2), carbon dioxide (CO2), organic acids, amino acids, and alcohols by different groups of bacteria. Some of these intermediate products (acetic acid, hydrogen, and carbon dioxide) can be directly used by methanogenic bacteria, but most of the organic acids and alcohol are decomposed into acetic acid, hydrogen, and carbon dioxide during acidogenesis. Only these products, as well as methanol, methylamine, and formate, can be transformed into carbon dioxide and methane (CH4) by methanogenic bacteria during the third and last step, methane formation.
A great advantage of anaerobic fermentation is the production of biogas that can be used as a source of energy. A major advantage of anaerobic digestion in comparison with aerobic composting is the ability of engineers to have total control over gaseous and liquid emissions, as well as having the potential to recover and use methane gas generated as the wastes degrade.